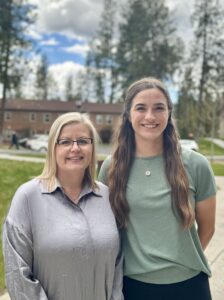
KERI RONEY & CORINNE VASILOFF
Keri: Class of 2024: I am a wife and a mother of two amazing children. After 15 years of being a CT technologist, I decided to pursue my dreams of becoming a doctor. I am graduating from Whitworth University with a B.S. in Biology. My next step is applying to medical school.
Corinne: Class of 2024. I am student-athlete at Whitworth pursuing my B.S in Biology with a minor in Philosophy. I am a captain and starting pitcher on the Whitworth Softball team and have been recognized as a second-team all-conference pitcher as well as an all-NWC scholar-athlete and CSC Academic All-District Athlete. I am graduating with honors alongside my twin brother, Nate, and will apply to medical school this summer.
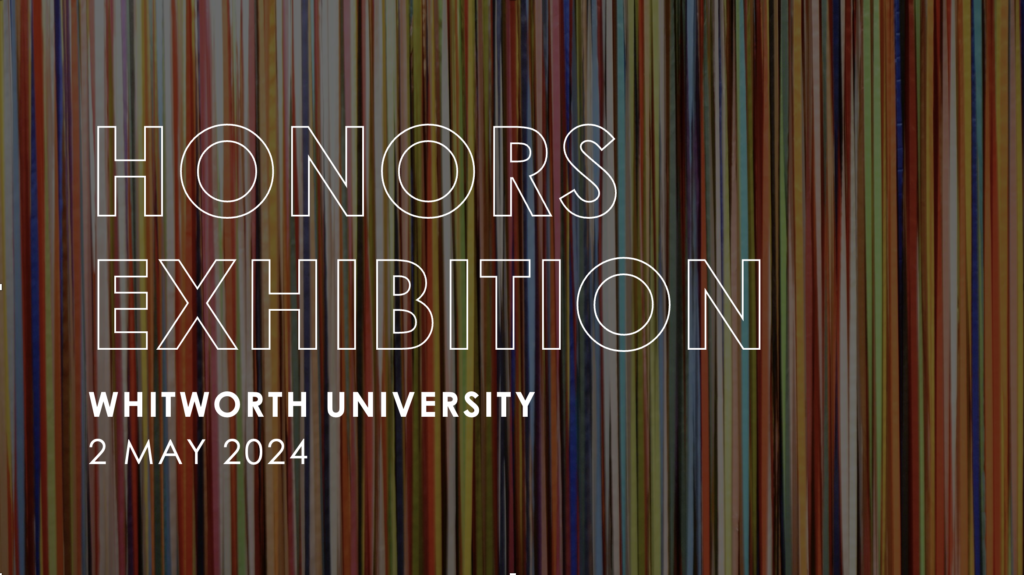
epigenetic and athletics: Preventing chronic illness through youth sports.
Epigenetics
Epigenetics refers to a relatively new, but vitally important, specialty in the field of genetics. It focuses on modifications made to DNA and related proteins that do not change the sequence of nucleotides but can change how they are expressed. Epigenetics can also be described as the interaction between the genome and environment, as many of these modifications are the result of external factors. Epigenetics is becoming increasingly important to a well-rounded understanding of genetics and how it affects health, but we are still in the early stages of understanding the scope of that effect. Epigenetics has been linked to specific physiological traits, including diseases related to the immune, metabolic, and cardiovascular systems, as well as psychiatric conditions like schizophrenia.1 There are factors that we do not fully understand that affect the process, like individual bias, cell-type specificity, and alterability during ontogenesis. Epigenetic research has already made it clear that additional focus should be placed on non-protein-coding regions because of the roles non-coding regions and miRNA have on regulation.1,2
Epigenetic modifications tend to affect DNA accessibility to transcription mechanisms, which affects gene expression patterns. One of the most studied types of epigenetics is methylation, the addition of a methyl group to DNA and other molecules. An increase in methylation generally means less transcription of downstream proteins because of reduced accessibility for proteins, but it can occasionally enhance expression by blocking silencing mechanisms.1 While newer research is examining the mechanisms and frequency of demethylation, methylation is generally stable, with life-long effects on the individual and the expression of the associated genes.
Methylation is studied on multiple levels. DNA methylation commonly involves the transfer of a methyl group to the fifth carbon pyrimidine ring of cytosine that precedes a guanine nucleotide (called a CpG sequence). It is catalyzed by a class of highly conserved DNA methyltransferase enzymes (DNMT).3 Methylated gene promoters have a limited ability to bind transcription factors, which reduces their expression levels. Current research also points to DNA methylation as being responsible for silencing the X Chromosome and parental imprinting, which is an important part of genetic inheritance. Methylation is also studied on a gene-specific global level, which looks more broadly at which genes demonstrate significant expression modification and how epigenetics mediate adaptations to environmental conditions. Methylation levels are not universal and differ across different tissues, even in the same individual,4 but it is frequently studied for quantitative measurement as a more accessible mark of epigenetic modifications.
Chromatin modifications are another common part of epigenetic modifications. More specifically, histone proteins are targets for covalent modifications such as methylation, acetylation, phosphorylation, and ubiquitination.1,5 To package genetic information into the nucleus, DNA winds around histones for protection, organization, and regulation. Because the DNA is wrapped around the histone, some genes are exposed to proteins more than others. Chromatin modifications are important in epigenetics because the chemical changes alter the proteins’ ability to attract and interact with DNA, which changes the levels of transcriptions for specific genes. With respect to histones, acetylation exposes DNA for transcription, whereas methylation tends to have activating and inactivating effects.1 DNA methylation and these chemical changes to chromatin-associated proteins are likely to interact to regulate gene expression, and there is evidence that epigenetics also affect the regulation of post-transcriptional splicing.1
Epigenetic inheritance means that some epigenetic modifications can be passed through generations of individuals. Most methylation is erased in a new zygote after fertilization or during early development. However, genomic imprinting is also related to DNA methylation inheritance. The paternal genome is demethylated after fertilization, but imprinted genes from the maternal genome are protected. These imprinted genes are very important in embryonic development, and irregular methylation patterns in imprinting regions can be lethal and have been associated with developmental disorders, metabolic diseases, and cancer.1,6
Just as epigenetic conservation is affected by development, epigenetics change throughout an individual’s life. There is a very low level of methylation at the neonatal stage, but the extent is partially dependent on the age of the parents (especially the father). The highest levels of age-associated methylation occur in childhood, puberty, and old age.4Between maturity and old age, a decrease in methylation is generally observed, as the machinery involved shows reduced capabilities. Interestingly, there seems to be greater variety in methylation with age, potentially caused by the increase of exposure to environmental factors throughout one’s life.4
There are two terms often used to describe the changes in epigenetics seen throughout aging. The first is Epigenetic drift, which refers to the tendency for increasing divergence in the epigenome over time. Epigenetic drift is affected by random methylation patterns and is therefore studied within an individual, but the changes are not common across separate individuals. The Epigenetic Clock, on the other hand, refers to changes in specific sites that are consistently related to time and aging, and are therefore common across individuals.4,7 Jones et al., (2015)4 states that while these terms have been used interchangeably in the past, newer research has demonstrated the importance of differentiating them.
The Relationship Between Athletics and the Epigenome
A phrase that seems to be repeated in both the world of medicine and athletics is “well it’s just genetics.” Some athletes have natural abilities and traits that set them up for success. One individual may have genes that promote their health, while another predisposes them to diseases. Athletic ability is undoubtedly influenced by genetics, but scientists are now looking more closely at the role played by molecular genetics and the epigenome. Heritable differences have been identified because of polygenic control (two or more genes interacting), which is generally associated with DNA sequences, but there is growing evidence about the influence of variable gene expression, which is more closely related to molecular genetics and epigenetics.1 A holistic understanding of the relationship between our DNA and physical performance will require a more in-depth study of the dynamic cellular network, instead of focusing only on genomics or even epigenomics.
Studies on physical performance tend to look at physiological components, like endurance capacity, maximal strength, power, and coordination, but it is also clear that an individual’s interaction with their environment is crucial to their performance capabilities. Many exercise physiologists have concluded that at least 50% of physical performance is dependent on external or controllable factors (rather than genetics) but that does not necessarily account for epigenetic changes.1 New research demonstrates that exercise influences the expression of genes related to muscle work, especially those involved in energy metabolism.3,8 These changes in expression level are the result of epigenetics, specifically histone modifications, DNA methylation, and miRNA production.9
While there is a lack of uniformity among research methods, studies have indicated that both acute and chronic exercise can affect epigenetic modifications.8 There is a wide variety in how studies are conducted, including types and measurement of exercise, species, types of tissue, global or gene-specific examinations, and offspring sex.8 Researchers are also looking for alternatives to Genome-wide Association Studies when looking specifically at the epigenome rather than genomic sequences. It is currently difficult to attribute specific physiological outcomes directly to epigenetic changes, but the field of epigenetics is continuing to develop, as is our understanding of how important it might be to fully understanding how genetics affect our health.
How Epigenetics and Exercise can Affect Health
It is widely known that the body adapts positively to exercise and physical activity, but those adaptations are complex, and we are still working towards a better understanding of the mechanisms that underlie multi-organ adaptation to exercise. The body reacts differently depending on the type of exercise. For example, Światowy et al. (2021)3 identifies that endurance exercise tends to result in increased oxidative capacity whereas resistance results in greater protein synthesis. Exercise can act as a preventative measure, reducing cardiovascular-associated mortality by 35% and decreasing risk for hypertension, hypercholesteremia, Type II Diabetes, and metabolic syndrome.8,7
Exercise causes changes in global methylation, which can affect gene expression in the long-term. It is typically studied in white blood cells but is also expected in skeletal muscles and adipose (fat) tissue. Epigenetic mechanisms in skeletal muscles have been shown to facilitate regrowth after injury, aiding in the recovery process.10Methylation patterns change rapidly depending on the intensity and duration of exercise. Światowy et al. (2021)3 noted significantly more global methylation with 26-30 minutes of exercise per day compared to individuals with less than 10 minutes a day. They also looked at how methylation patterns changed with age. As noted above, global methylation levels decrease with age, and this hypomethylation has been associated with genomic instability. Epigenetic changes that result from exercise may extend life expectancy by maintaining methylation patterns.3,9
Gene methylation from exercise has also been studied in specific tissues. When examining muscle tissue, even acute exercise was shown to induce changes in the levels of methylation.3 Consistent physical exercise over a period of time caused muscles to adapt more strongly and modify the methylation profile on a larger scale. Światowy et al. (2021)3 describes this as reprograming the organism through methylation, building Epigenetic Memory, so that the body reacts more strongly through methylation to a secondary effort. The transient nature of adaptation through exercise-related methylation supports the importance of consistency, duration, and intensity of exercise.8 Epigenetic modifications can also reduce the risk of type II diabetes, although the disease may resist the changes. More generally, epigenetic changes induce differences in expression of the genes involved in muscle metabolism.3 Polygenic control also becomes important in muscle tissue adaptation because multigene interactions can multiply the impact of methylation.3
Exercise-induced methylation has also been seen in fat, blood cells, and diseased tissues.3,9 In blood cells specifically, exercise affects a body’s inflammatory response, but that may be limited by age. Diseased tissues also show modifications related to epigenetics. They found that exercise-induced methylation occurs in promoters for genes that are important in cancer risk and development. Interestingly, those studies also show that the intensity and duration of exercise determines how much of an effect there is on specific kinds of cancer. For example, there was a decreased risk of breast cancer due to global methylation from long-term exercise, specifically because methylation of the relevant gene is highly stable. Methylation related to aerobic exercise was also found to be beneficial for brain development and function.3
Potential inheritance was also studied for exercise-related modifications to the epigenome. Axsom and Libonati (2019)8 found altered methylation patterns, miRNA levels, and functional outcomes in offspring of exercising parents. Both global DNA and promoter-region (non-coding) methylation differed in exercise-offspring. The genes that seem to be the most affected are involved in metabolism. mRNA transcript levels indicate changes in oxidative metabolism and the regulation of growth in offspring. Prenatal exercise showed benefits to the exercising parents and the offspring. Maternal and paternal physical activity pre-conception showed improvements in the offspring’s glucose tolerance and paternal exercise was associated with more favorable birth weight.8 This indicated that in addition to the epigenome’s potential heritability, epigenetic modifications may also be more directly involved in prenatal and neonatal development.
Conclusion
Epigenetics can be described as the interaction between external factors and genetics. It does not edit the DNA sequence itself but changes the accessibility of genes through methylation and chromatin modifications. These mechanisms, along with epigenetic changes to non-coding RNA, regulate the levels of expression for specific genes. There is a known relationship between exercise and epigenetic changes throughout the body. Further research is needed to fully understand the impact of those changes on overall health, but it is highly likely that exercise-induced epigenetic modifications could have long-term effects that might even be passed down to future generations. Physical activity has been shown to prevent disease, maintain epigenetic stability through aging, and build epigenetic memory. Because the epigenome is especially transient through childhood and puberty, consistent physical activity during that time could have significant life-long benefits.
References
- Ehlert T, Simon P, Moser DA. Epigenetics in sports. Sports Medicine. 2013;43(2):93-110. https://doi:10.1007/s40279-012-0012-y
- Ordovás JM, Smith CE. Epigenetics and cardiovascular disease. Nature Reviews Cardiology. 2010;7(9):510-519. https://doi:10.1038/nrcardio.2010.104
- Światowy WJ, Drzewiecka H, Kliber M, et al. Physical activity and DNA methylation in humans. International Journal of Molecular Sciences. 2021;22(23):12989. https://doi:10.3390/ijms222312989
- Jones MJ, Goodman SJ, Kobor MS. DNA methylation and healthy human aging. Aging Cell. 2015;14(6):924-932.https://doi:10.1111/acel.12349
- Martin C, Zhang Y. Mechanisms of epigenetic inheritance. Current Opinion in Cell Biology. 2007;19(3):266-272. https://doi:10.1016/j.ceb.2007.04.002
- Kandi V, Vadakedath S. Effect of DNA methylation in various diseases and the probable protective role of nutrition: A mini-review. Published online August 24, 2015. https://doi:10.7759/cureus.309
- Quach A, Levine ME, Tanaka T, et al. Epigenetic clock analysis of diet, exercise, education, and lifestyle factors. Aging (Albany NY). 2017;9(2):419-446. https://doi:10.18632/aging.101168
- Axsom JE, Libonati JR. Impact of parental exercise on epigenetic modifications inherited by offspring: A systematic review. Physiological Reports. 2019;7(22). https://doi:10.14814/phy2.14287
- Zimmer P, Schenk A, Bloch W. Chapter 29 – Epigenetics in Exercise Science and Sports Medicine. In: Medical Epigenetics. Academic Press; 2016:515-530.
- Tarnowski M, Tomasiak P, Tkacz M, Zgutka K, Piotrowska K. Epigenetic Alterations in Sports-Related Injuries. Genes (Basel). 2022;13(8):1471. Published 2022 Aug 17. https://doi:10.3390/genes13081471